Heel-deep in it
Nuclear power produces large amounts of toxic waste.
It produces toxic waste but so do many industrial processes. Whether it can be qualified as being produced large amounts is debatable. Because nuclear fuel has such a high energy density, very little of it is required to produce a given output. Since little fuel goes in, it follows that little waste can come out. In fact, high level waste without reprocessing, which accounts for 95% of the total radioactivity of radwaste, is produced at a rate of around 1m³ per gigawatt-year for a typical light water reactor.
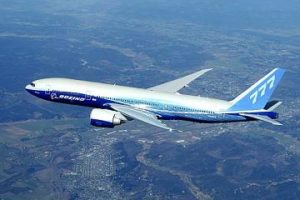
Figure 1- Boeing 777-200LR medium capacity airliner, the platform for the upcoming 777 freighter. (Image courtesy BCA)
For an idea of what this means, consider the American nuclear power output for 2005, which amounted to around 90 GW.yr. The volume of high level waste as spent fuel produced is therefore around 90m³. This means that almost a decades worth of high level waste from the entire American civil reactor fleet could fit into a single Boeing 777 freighter such as one based on the medium capacity aircraft shown in figure 1 (getting the aircraft airborne with that load is another matter however). So Air France’s order for 5 Boeing 777Fs is sufficient to store the spent fuel produced by the US reactor fleet over its entire lifetime.
Of course HLW would not be crammed into aircraft in this fashion, since it requires shielding and space for heat dissipation (after first removal from the reactor, spent fuel is placed into cooling pools for a few years while the most radioactive fission products decay away). It is simply an illustration of scale. Future reactors, with higher burnups, will reduce this further.
Solid waste from coal burning, which includes the permanently toxic metals mercury, arsenic and selenium, is produced a thousand times faster. This cannot, by any standard, be characterised as fit for human consumption either. It is however, not subject to the same stringent controls and accounting as nuclear waste is. Because of its large quantity, to impose similarly proportionate controls would be prohibitive. In addition, fossil fuel burning also releases large gaseous emissions into the atmosphere including sulphur dioxide and nitrogen oxides as well as particulates.
Nuclear waste remains toxic for tens or hundreds of thousands of years.
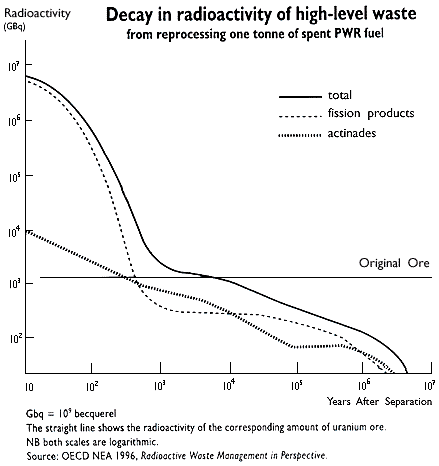
Figure 2- Decay curve for spent PWR fuel. (Image courtesy of the WNA)
Tens or hundreds of thousands? No. Unreprocessed spent fuel will have decayed to below the activity of the original uranium ore in under ten thousand years. Figure 2 shows the generic decay curve for spent PWR fuel. Figures such as 50,000, 100,000, or sometimes millions of years get tossed around, but they are more likely to have originated from a random number generator (hyperbole always involves randomly large numbers). The radioactivity of the original uranium ore is generally used as a benchmark since it is what we would be dealing with if it were not for nuclear power. Uranium ore is also not especially radioactive and so represents a relativity benign level of activity.
It should also be remembered that radioisotopes decay exponentially. Spent fuel does not remain glowing green for ten thousand years at which point it stops being significantly radioactive. Throughout the millennia, it is continually decaying, becoming less and less radioactive, less and less hazardous, as time goes on. And because the decay is exponential, most of it happens in the early stages so that the majority of time period is spent at a significantly lower level of activity than at initial disposal and in fact a level that is not especially hazardous at all. Compare this to those mercury and arsenic solid wastes from coal burning, which will be just as hazardous in ten thousand years as they are today.
However, it is of course the case that regulatory agencies are never happy with that, particularly when nuclear power comes into the mix, which is why agencies like the EPA demand that any methods of spent fuel disposal be able to contain the material for at least 10,000 years, even though it will have stopped being a significant hazard long before this. It should be remembered that regulatory agencies are always over cautious about things and so their criteria do not define the limits of safety. Safety comes well before their criteria.
CLOSING THE CYCLE
Figure 3- The changes in the composition of tonne of fresh LWR fuel after 3 years of irradiation. (Image courtesy of the WNA based on information from June 1977 edition of Scientific American)
Almost ten thousand years still sound too long? Fair enough. That is not the whole story. Figure 3 shows the typical composition of fresh and spent fuel from a Generation II LWR. Most reactors are light water reactors, which use uranium 3-4% enriched with uranium-235. Since uranium-235 is the main isotopes, which is burnt in the reactor, this means that spent fuel is around 3-4% fission products, while the rest is unburned actinides, mostly the uranium-238, which went in, but also some other actinides, including plutonium and also americium, which may find its way into your smoke alarm. Burning them is more difficult than with uranium-235, but it can be done.
The key is that it is these actinides, which give the hazard of spent fuel its longevity. Fission products, though highly radioactive at first, also decay much more quickly. By increasing the proportion of short lived fission products and reducing the proportion of long lived actinides, the waste becomes benign much more quickly. What is comes down to is the need for greater burnup of fuel. The more a mass of fuel is irradiated, the less actinides will remain. It also has the benefit of reducing the amount of fuel needed to produce a given amount of energy and so the quantity of waste overall is reduced.
The simplest way to increase burnup, is through DUPIC, Direct Use of spent PWR fuel In CANDUs. Because CANDUs are heavy water moderated, they have a much better neutron economy and so can be critical on this spent fuel, even when LWRs cannot. The only processing needed is purely physical not chemical. The spent PWR fuel must be fabricated into CANDU fuel bundles.
For a more thorough use of actinides, though, they must be separated from the rest of the spent fuel. This is done through reprocessing. After some time in the cooling pools, waiting for the most radioactive fission products to decay away, the spent fuel is then reprocessed, which involves separating out the actinides for recycling. Since 97% of the spent fuel is actinides, this reduces the waste quantity significantly and hugely improves the yield from the natural uranium resource.
Plutonium is easy enough to use in mixed oxide (MOX) fuel for specifically configured reactors. CANDUs are of course available for burning other actinides in certain forms of fuel. Future methods for burning minor actinides include the use of fast reactors, which can burn a wide variety of actinide compositions, and accelerator driven reactors, which can burn an even greater variety.
Fast reactors can be configured to either burn the core of plutonium and other actinides, fast burner reactors, or, with the inclusion of a blanket of fertile material, additionally breed more fissile material, fast breeder reactors. Most Generation IV concepts are fast reactors incorporating widespread actinide recycling for efficient use of uranium resources. Accelerator driven reactors use a proton accelerator to sustain a fission reactor actively without the need for making the reactor critical.
This is closing the cycle.
As all actinides get burnt or used for other purposes, this leaves on fission products. Those, which are not extracted for other purposes, are treated and incorporated in borosilicate glass, a process known as vitrification. This renders the radioactive material immobilised and contained sufficiently for many millennia. This is more than sufficient because fission products, with their shorter half-lives require only six hundred years to decay to the activity of the original uranium ore as shown in figure 2.
The long term result of the nuclear fuel cycle is to lead to a reduction in radioactivity. Slightly radioactive ore is removed and replaced by material, which while significantly more radioactive at first, after a short while becomes less radioactive than what would have been there had the ore not been removed.
What effects does this have on the volume of waste?
Figure 4- A silo of canisters holding vitrified waste at Sellafield, UK. (Image courtesy of the WNA)
With full actinide recycle the volume of raw waste is reduced thirty fold. However, a lot of glass is introduced into the mix and the vitrified block is placed in canisters such as the ones shown in figure 4, so the end effect is an increase in the volume of high level waste for disposal. A gigawatt-year of canisters of vitrified fission products from a Generation II LWR, will typically be around 2-3m³ in volume. So we may need up to fifteen 777F’s every half-century. This is still a minute quantity of waste on the industrial scale for even one year- or a week in some sectors- let alone half a century.
There is no way to dispose of HLW, be it actinides or fission products no matter what the term.
The obstacles to proper waste management for the either the open cycle or the closed cycle are purely political. Geological disposal uses a multiple barrier system, starting with immobilisation, then providing proper containment for the immobilised waste, then placing it in a stable geological formation.
Vitrification can be used to immobilise all the hazardous materials, although since uranium and plutonium are so valuable, and vitrifying them makes them irretrievable, there is quite sensibly a reluctance to do this. Synroc is another immobilisation technology under development, which secures the material inside a crystalline matrix. This is somewhat similar to the way the natural reactor at Oklo in Gabon contained its fission products and plutonium when the rich vein of uranium was moderated to criticality by seeping ground water two billion years ago.
The immobilised waste is then placed in stainless steel containers and concrete dry casks. The containers are the buried in an impermeable clay within a specially selected underground repository, chosen for its geological stability and isolation from ground water. It would take millions of years for the containment measures to be eroded down by water, even if the climate and geology were to suddenly change allowing water into the repository, and then have the radioactive material transported out into the environment. This is more than sufficient because within ten thousand years, even unreprocessed spent fuel, which really should not be buried anyway, will be no more harmful than granite.
The problem with long term geological disposal is not technical, it is political.
Is this really the legacy we want to leave our children?
Pathetic appeals to emotion aside, we, and previous generations, have left many waste dumps far greater in size and far less contained. Spent nuclear fuel disposal is the most comprehensive and carefully planned of all waste disposal practises. It all the other forms of waste disposal that are closer to being a serious negative legacy, not a relatively small quantity of a diminishingly hazardous material, buried deep underground beneath many layers of isolation.
The Yucca Mountain fiasco, so far 8 years late and counting, demonstrates how difficult it is to dispose of spent fuel.
It demonstrates how difficult it is to get passed political opposition, with all the frivolous lawsuits from the State of Nevada and campaigns based on FUD. Yucca Mountain incorporates multiple barriers from the casks encasing the spent fuel to the geological stability of the area used. The case against the environmental security of Yucca Mountain is based on manufacturing an improbable set of circumstances over an excessive length of time.
First, it would require the climate to change making the now desert region turn into an area frequented by water and the geology would need to change to allow the water to permeate the currently impermeable rock so it comes into contact with the casks. The water would then need to erode down the concrete, which would take a great deal of time. Then it would have to eat its way through the stainless steel containers. Finally it would have to dissolve the spent fuel and whatever material is used to immobilise it. Then the material must be transported out of the repository to inhabited areas.
And the deadline for all this, climatic and geological shifts, erosion through multiple layers of hardened materials, and transportation over long distances, is 10,000 years. Normally, it takes many millions of years for something of this scale to happen and that is in circumstances where the barriers were not designed to be resistant to these things. There was no sophisticated containment for the Oklo reactor and yet no significant movement has happened even after billions of years.
Yet the originally planned opening date for Yucca Mountain has come and gone by 8 years and no sign of completion is on the horizon. It was not a subsidy that was supposed to pay for this. American nuclear utilities pay 0.1c/kWh towards a spent fuel disposal fund, which would be used by the government to provide the service. The utilities continue to have to store spent fuel on their plant sites at their own cost because of the delays (though it should be noted how remarkable it is that several decades worth of waste can still be stored in a relatively tiny space on site). The incompetence of the government at fulfilling their part of the business transaction has prompted the utilities to actually sue for damages, by wasting the money they have paid into the spent fuel fund while simultaneously forcing them to pay extra for continued interim storage.
That said, many people do not think Yucca Mountain is ideal, because it is seen as wasteful since it disposes of perfectly useful energy resources. Closing the cycle with fast reactors and reprocessing is the better solution for waste. The DoE policy of Yucca Mountain is based around these practises still being outlawed in the United States.
Low level waste and intermediate level waste are the real waste problem.
Low level waste comprises 90% of all radwaste but only 1% of the radioactivity. Most of it is clothes and rags contaminated with small quantities of radionuclides. They do not represent a large hazard and some are only considered radwaste at all because of overprotective attitude of the regulatory authorities when it comes to radiation. Comparable levels of activity in materials not used by the nuclear industry do not receive the same treatment (for example, materials with natural radioactivity must be treated as LLW by the nuclear industry, but will be treated normally by other ones). Low level waste can be buried in shallow landfills such as Drigg at Sellafield. The chemical hazards from all manner of other wastes from other industries represent as much or more of a threat than low level wastes from the nuclear industry.
Intermediate level waste is more of an issue. It comprises some reprocessing wastes and some activated reactor components salvaged during decommissioning. It does require some shielding and longer lived isotopes are buried more deeply. Lead is sufficient for this. Due to the low levels of radioactivity compared to high level waste, there is no technical problem in disposing of this properly. Some highly toxic chemicals wastes such as cyanides represent a greater hazard, but are less heavily regulated. Intermediate level waste comprises 7% of all rad waste so it does not represent a problem of scale.
LLW and ILW do not possess any kind of extraordinary hazard and they are not produced in any unmanageable quantities on the industrial level. There disposal is equivalent in difficulty to a plethora